5.11.2021
Will we one day image oceans on another Earth? Will we ever see the signatures of the first black holes, or map out the nearly invisible cosmic web? Will we detect the origins of planets and life itself? These are among the most pressing questions in astronomy today — and the answers lie within our reach.
Astronomers are proposing four flagship mission concepts: HabEx, LUVOIR, Lynx, and Origins
NASA
In the next few weeks, a high-powered group of astronomers spanning a range of disciplines will publish guidelines that will set the priorities for the next decade of astronomy. Selected by the National Academy of Sciences, this group is considering four flagship space telescope missions — any one of which could change the face of astronomy — as well as 10 smaller probe missions. The astronomers will set down a vision of the future in a report called the decadal survey.
Decadal surveys have been setting the tone for space-based astronomy for the past 50 years. So far at least, any space mission that received highest priority in a given decadal eventually made it to the launch pad. That list includes the Hubble Space Telescope, which received priority in the 1972 report and launched in 1990; the Chandra X-ray Observatory, which received priority in 1982 and launched in 1999; and the Spitzer Space Telescope, which received priority in 1991 and launched in 2003.
The James Webb Space Telescope (JWST) is up next, having been prioritized in 2000, then the Nancy Grace Roman Telescope (formerly known as Wide Field Infrared Survey Telescope, or WFIRST), which received first place in the 2010 survey. Neither mission has launched yet. While they will likely still make it to space, James Webb in particular has extracted a political cost with numerous delays and budget overruns. That’s why in 2016 NASA started funding detailed studies — including everything from costing models to work breakdown structures — for four “large mission” concepts: HabEx, LUVOIR, Lynx, and the Origins Space Telescope (listed in alphabetical order).
With these studies, NASA hopes to stave off another JWST-like situation. “Each of these four studies at this point in time has done so much more than any large mission concept prior to a decadal survey in history,” says LUVOIR team member John O’Meara (W. M. Keck Observatory).
“Large” is almost a misnomer for these concepts; “transformational” might be a more appropriate choice of words. The potential that any one of these observatories carries should send a thrill down the spine of any astronomer. From direct imaging of Earth-size planets to detecting the first black holes at the dawn of time, these missions promise tremendous gains for science.
Any one of these missions will need at least several billion dollars to design, build, and launch. So it’s fair to say that the decadal committee will only grant priority to one of these missions — assuming they decide a flagship is even necessary. Read on to see what transformations are in store.
MISSION CONCEPTS IN A NUTSHELL
Mission | Primary Mirror | Wavelengths Covered | Instruments | Primary Innovation | Science Goals |
HabEx | 4 m (off-axis secondary) |
Ultraviolet, visible, near-infrared |
|
52-meter starshade flying 76,000 km from telescope |
|
LUVOIR | 8–15 m | Ultraviolet, visible, near-infrared |
|
Unprecedentedly stable large mirror |
|
Lynx | 3 m | X-rays |
|
Thousands of thin X-ray-reflecting mirrors nested inside each other |
|
Origins | 5.9 m | Mid-/far-infrared |
|
Ultra-cold telescope (4.5 K) and next-generation detectors |
|
HABEX: HUBBLE 2.0
It’s easy to think of HabEx as a next-generation Hubble Space Telescope. With a 4-meter primary mirror — twice the size of Hubble’s — HabEx will detect near-infrared, visible, and ultraviolet light. But here’s where it really stands apart: Outfitted with both a coronagraph and a 52-meter foldable starshade, HabEx is set up to directly image small exoplanets. The coronagraph is inside the spacecraft, but the starshade would fly separately, some 76,000 kilometers from the telescope.
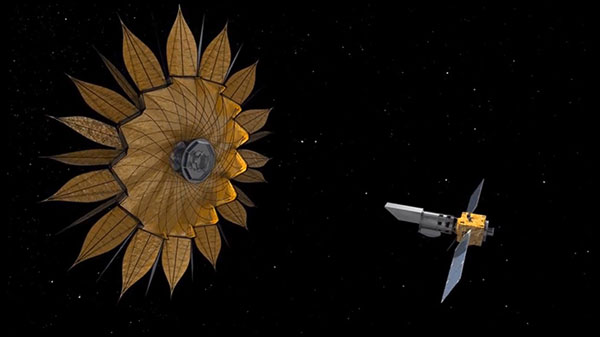
HabEx / NASA
The ultimate, spine-tingling goal of this outlandish setup: Find and study another Earth. HabEx will image and characterize a dozen rocky, Earth(ish)-size planets around Sun-like stars. The mission’s instrument suite is designed to look for the signs of habitable atmospheres and chemical signs of life called biosignatures. Scientifically speaking, that includes the ability to measure oxygen, carbon dioxide, and methane absorption features in these planets’ atmospheres. HabEx could even detect starlight glinting off oceans.
Part of what makes all of this possible also represents the trickiest part of the mission. A starshade (aka “space daisy”) has never flown before, though the concept has been studied since the 1990s. The reason it’s needed is because while a coronagraph inside the telescope can blocks much of a host star’s light, some starlight inevitably scatters back into the field of view. A starshade prevents this backscatter, enabling the detection of smaller and fainter planets.
The challenges are twofold: First, the starshade must fly on its own, outside the telescope, using its own propulsion system to not only stay aligned with the telescope but also to slew to new targets. Second, the starshade is huge — the size of two baseball diamonds — so it must fold for launch and then unfold in space, with little forgiveness for errors. Imaging a dozen exo-Earths with a 4-meter telescope requires that the starshade and coronagraph work together.

HabEx / NASA
That said, HabEx isn’t 100% reliant on the starshade: The team is considering nine total configurations. Alternatives include replacing the 4-meter mirror with a 3.2-meter, segmented mirror, as well as the option of not including a coronagraph, starshade, or both.
“Our preferred architecture is the most expensive one, with the most technologies that need to be developed,” says Gaudi, co-chair of the HabEx team. “All the other architectures are less expensive and require fewer technologies to enable the science, but of course they also can't do as much science.”
Despite its name, though, there’s much more to HabEx than exoplanets. Half of the telescope’s observing time will be dedicated to general ventures, enabling astronomers to explore everything from the missing matter of the universe to the yet-unseen aurora of the ice giants Uranus and Neptune. In a sense, it’ll do much of what Hubble does now. But HabEx isn’t just replacing Hubble. With a primary mirror twice the size of Hubble’s, HabEx will have four times the photon-gathering power, as well as improved camera and spectrograph technologies. HabEx will see more and farther than Hubble ever could.
LUVOIR: BIGGER, BROADER & BOMBASTIC
If HabEx is the next-generation Hubble, then the Large UV/Optical/IR Surveyor (LUVOIR) could be compared to Hubble bitten by a radioactive spider. LUVOIR’s superpower is its segmented 15-meter mirror, which would unfold, JWST-style, after launch. The team is also presenting an alternative configuration with a smaller, but still-unprecedented, 8-meter mirror.
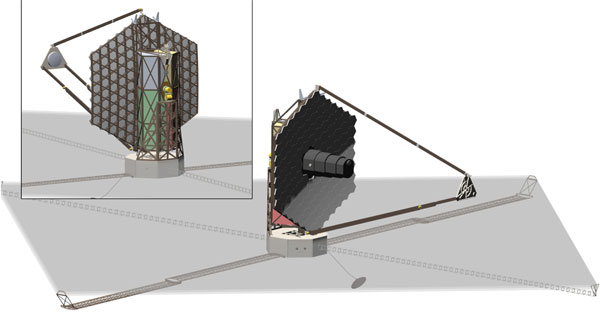
LUVOIR / NASA
Like the other large mission concepts — and like Webb and Roman — LUVOIR would orbit the Sun at the L2 Lagrange point, 1.5 million kilometers anti-sunward from Earth. But unlike the other missions, LUVOIR is designed for astronauts to service it, which provides a safety net, albeit an expensive one, as well as opportunities to replace and upgrade instruments.
Servicing is also necessary if the mission is to go beyond 10 years. To keep the spacecraft from tumbling in space, also known as station keeping, it will fire microthrusters rather than rely on the kind of reaction wheels that have failed on Hubble and Kepler. But with a 15-meter mirror and an 80-meter shade to protect it from the Sun’s heat, LUVOIR is going to go through its fuel quickly, limiting its lifetime unless it’s refueled.
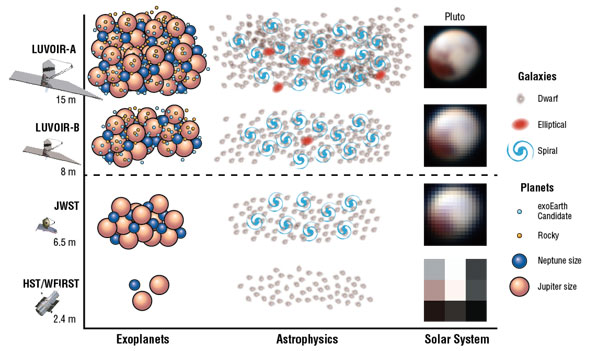
NASA / New Horizons / J. Friedlander & T. B.
Griswold (NASA GSFC)
LUVOIR’s driving goal is to directly image exo-Earths — not only a dozen, like HabEx, but a hundred of them. Unlike HabEx, a starshade won’t accompany LUVOIR. However, its big mirror, finely tuned picometer-level stability for the telescope’s optics, and a next-gen coronagraph should more than make up the sensitivity needed to answer the question: Are habitable planets — and life itself — common in our universe?
“I can imagine two scenarios that are very profound,” says O’Meara. “One is that we survey 100 Earth-like planets around Sun-like stars in the habitable zone and we find nothing. And the other is we survey planets in the habitable zone and we find that life is abundant.”
Team member Aki Roberge (NASA Goddard) explains that a large sample size is crucial to making the distinction. “HabEx can’t do this, as they will well admit, because their sample size is too small. LUVOIR's larger telescope aperture enables our larger sample size.”
Gaudi agrees, to a point: “HabEx would be the ‘first step’ in trying understand this question. LUVOIR is the ‘giant leap’ to try to not only answer this question, but provide a robust numerical answer to it.”
“The great thing about HabEx and LUVOIR,” Gaudi adds, “is that we have provided the community a continuum of options that can be responsive to whatever risk posture we (as a society) are willing to adopt.”
Exoplanets are far from the only revolutionary science LUVOIR would do. The telescope will reach a magnitude of 33–34 — fainter than anything that could ever be seen from Earth due to our sky’s 30th-magnitude sky glow, and fainter even than Hubble’s 31st-magnitude limit. “We don't even have a cosmological simulation that goes that faint,” O’Meara says. “It's absolutely undiscovered country.”
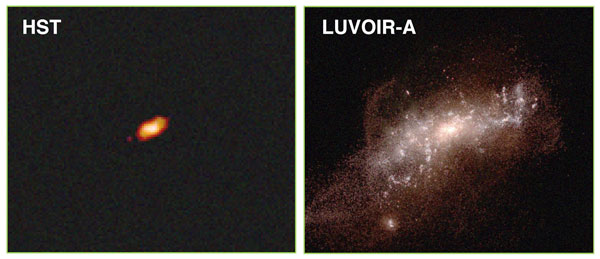
G. Snyder (STScI)
Probing at these levels will likely reveal dwarf galaxies throughout the universe, which current telescopes can’t detect. “With something like LUVOIR,” O’Meara notes, “we’d be able to see a 100 solar mass dwarf galaxy 10 billion light-years away.” Such a powerful telescope could also, for the first time, map the hot gas around galaxies that we know is there but can’t yet directly image.
But with great power comes a great price tag. “LUVOIR is arguably the most ambitious of the four missions,” O’Meara says. “That carries a cost with it, of course. Will LUVOIR be more than $5 billion? Yes.”
“It depends on how ambitious your goals want to be and what type of science you want to do,” O’Meara adds. “It’s the most exciting thing I've worked on in my career to know that we can build this thing. We have the capability and we have the people, all we need is the courage to do it.”
LYNX: NEXT-GEN X-RAY VISION
The next mission concept takes us out of the visible and near-visible range and into the X-ray regime. X-rays come from the hottest and most energetic processes in the universe, shedding light on gas-devouring black holes, exploding stars, and the hot gas teeming between galaxies. And Earth’s atmosphere blocks them completely — we didn’t even know cosmic X-rays existed until the Space Age.
Enter Lynx. Named for the keen-sighted feline that in many traditions is thought to see through to the true nature of things, this mission concept represents a leap of improvement over existing and near-future X-ray telescopes.
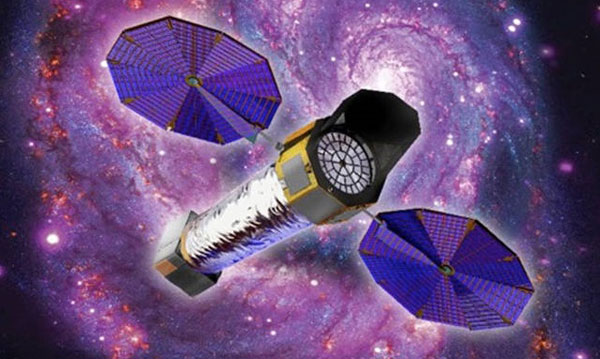
NASA / MSFC
The very high-energy nature that makes the photons Lynx will capture so illustrative also makes them hard to focus. After all, X-rays generally pass through material rather than reflect off of it. In order for X-rays to come to a focus, they must first reflect at grazing incidence angles off of a highly polished mirror, like smooth pebbles off a still pond. NASA’s Chandra X-ray Observatory has four such mirrors, nested inside each other like Russian dolls to capture sub-arcsecond X-ray images. XMM-Newton, the European Space Agency’s X-ray satellite, carries 58 nested mirrors in each of its three telescopes.
Lynx easily surpasses them all. “It will carry the most extraordinary X-ray mirror ever created, a 37,000-segment beautiful chandelier of highly polished silicon,” says team member Grant Tremblay (Center for Astrophysics, Harvard & Smithsonian). These silicon pieces will be stacked into 611 modules, and those will be combined into semispherical shells.
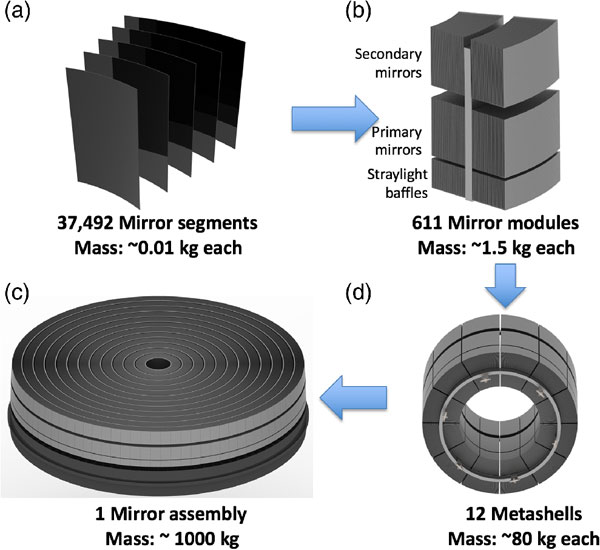
Zhang et al. / Journal of Astronomical Telescopes, Instruments, and Systems 2019
This mirror assembly is the mission’s keystone. By collecting oodles more photons, the telescope will achieve 100 times Chandra’s sensitivity, a feat that will enable it to see the first black holes at the dawn of time. Astronomers have long pondered how black holes at the centers of galaxies could have grown to millions of solar masses only a billion years after the Big Bang. Lynx will be able to probe 500 million years earlier, seeing the seeds of these supermassive black holes. The data it collects will help astronomers determined how these black holes formed and grew.
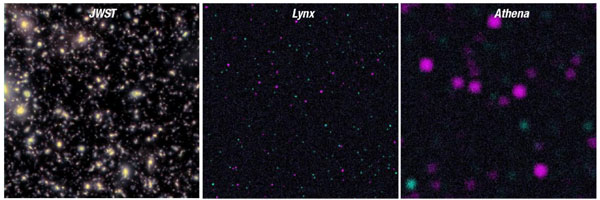
Lynx / NASA
Lynx will also survey the sky 800 times faster than Chandra can, with a field of view 22 arcminutes on a side and sub-arcsecond imaging across most of that field. Combined with its sensitivity and spectral resolution, this survey speed will help Lynx map the nigh-invisible hot gas that surrounds galaxies, permeates galaxy clusters, and outlines the cosmic web. This hot gas accounts for most of the universe’s “regular” (i.e., not dark) matter and underlies its structure, explains Lynx team chair Alexey Vikhlinin (Center for Astrophysics, Harvard & Smithsonian). While understanding that structure has long been the provenance of cosmological simulations, with Lynx we’ll obtain a real picture of it.
“An image of the cosmic web would be one of the most transformational images, like Earthrise, like the [Event Horizon Telescope] image,” Tremblay adds. “A single jpg that transforms everything.”
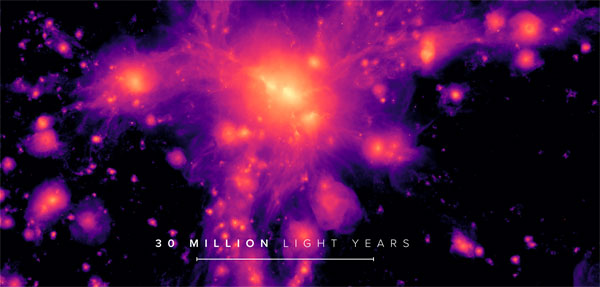
Lynx / NASA
Of all the missions, Lynx has the advantage of bringing groundbreaking science with the lowest need for new technology. The mirror assembly represents the mission’s primary technological advance; the rest of the spacecraft is basically the same as Chandra, which launched in 1999.
“No terrifying unfolding sunshield, no starshade which is technically a separate spacecraft that you're now flying at 80,000 km from your other telescope,” Tremblay says. “It's a tested, proven design. We take out Chandra's eye and we replace it with the most incredibly powerful eye you can ever imagine.”
The mirror itself is the most expensive component, but hundreds of pieces have already been built to date. “They now have pay stubs and invoices to prove how much it costs; it's not going to break the bank,” says Vikhlinin. “We will meet [NASA Astrophysics Division Director] Paul Hertz's guidance to be under $5 billion. And without compromises.”
ORIGINS SPACE TELESCOPE: ANSWERING "HOW DID WE GET HERE?"
Last but by no means least is the space telescope, dubbed Origins for short, that’s set to unveil a barely explored window on the sky. Far-infrared light probes the dust and gas between the stars, planet formation, and just-merged galaxies still shrouded in the debris of their collision. But, like X-rays, collecting these infrared photons requires rising above Earth’s atmosphere.
“The term ‘infrared’ encompasses near-, mid-, and far-infrared, each of which is about as expansive as visible or ultraviolet light on their own,” says Origins team member Cara Battersby (University of Connecticut). “While there is some very important overlap with JWST, Origins explores the universe in a wavelength range (far-IR) that has barely been tapped and is teeming with possibility.”
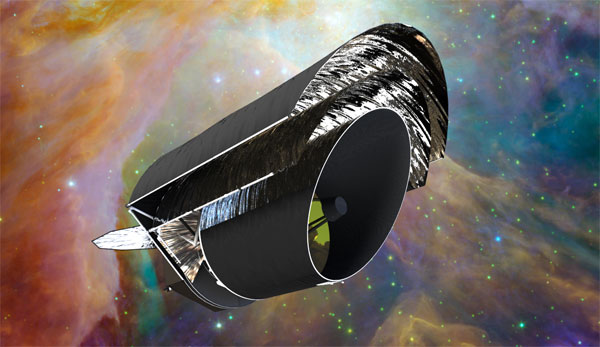
Origins Space Telescope / NASA
NASA’s Spitzer Space Telescope and the European Space Agency’s Herschel Space Observatory were the last to probe this long-wavelength regime. Herschel observed the far-infrared sky from 2009 to 2013, and while Spitzer is still operating, it’s observing in a “warm” mode that limits its capabilities. Origins promises a 1,000-fold increase of sensitivity over these observatories. “The advance offered by the Origins Space Telescope is akin to that from the naked eye to humanity’s first telescope,” Battersby says.
Like Lynx (which follows on Chandra and is structured much the same), Origins is the successor to Spitzer and relies on its tried-and-proven architecture. While Lynx provides a new-and-improved mirror, the technological advance Origins offers is its detectors. The mission team is still developing two types of far-infrared technologies — transition-edge sensor bolometers and kinetic inductance detectors. One of these technologies will ultimately selected for use in the telescope. A new mid-infrared detector is also under development.
Furthering the detectors’ capabilities will be the telescope’s ultra-cold temperature, just 4.5 degrees above absolute zero. “When a telescope is warmer than 4.5 K, the emission from the telescope is higher than the background, making it harder to see the universe,” says Origins team chair Margaret Meixner (Space Telescope Science Institute). “It’s like observing at optical wavelengths during the daytime: you can’t easily see the stars because of all the scattered sunlight. Origins, with a 4.5 K telescope, is reclaiming the ‘night sky’ in the far-infrared.”
The goal of investigating this relatively unexplored part of the spectrum is to look at our — you guessed it — origins: the birth of stars and galaxies, the beginnings of planets, and the ingredients necessary to create life itself. Carl Sagan famously declared, “We're made of star stuff,” and Origins will explore that stuff directly.
“I am most interested in the life cycle of dust over cosmic time,” Meixner explains. “How did dust form in dying stars, mix into the interstellar medium, and assist in the formation of the next generation of stars?”
Because of the nature of the expanding universe, the long wavelengths to which Origins is sensitive enable it to explore further into our cosmic past than current and upcoming telescopes can — back to the era of the first stars, dubbed the Epoch of Reionization.
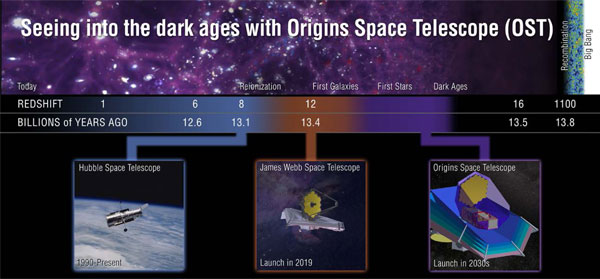
Origins Space Telescope / NASA
Like HabEx and LUVOIR, Origins will also have a crucial role to play in exoplanet exploration. With mid-infrared spectroscopy, the mission will be able to detect biosignatures on terrestrial planets at an Earth-like distance from their host stars. “Origins would operate over wavelengths at which potentially habitable planets emit radiation,” says team member Tiffany Kataria (NASA JPL). “Temperature measurements, along with measurements of biosignature molecules, would help us to determine if a planet is indeed habitable.”
The biggest draw, though, may be the unknown. “Whenever humanity has looked at a new wavelength range with such an increase in sensitivity, we have seen amazing new things,” Battersby says. “The science we don’t yet know is what I find most tantalizing.”
ONWARD AND UPWARD
It’s not quite fair to say that these mission concepts are competing against each other. After all, as Seager reminds us, “The decadal survey is under no obligation to choose one of the four.” After the bloated JWST mission, will the scientific community choose to greenlight another ambitious “Greater Observatory” — or press pause on flagship development?
“That is my biggest fear,” says O’Meara. “If we as a community say it's impossible for us to learn from our mistakes, it's impossible for us to build a flagship, then we're kind-of giving up on ambition. I think that would be very sad for astronomy if we did so. . . . It would be very bad for the country, because we would be giving up on truly ambitious leadership in space.”
We’ll have to wait and see. Astronomers everywhere will be opening the Astro2020 Decadal Survey late this summer, looking first and foremost at the survey’s number one priority for the next 10 years. Tremblay notes with a laugh, “That’s the big, top-level item that many bottles of Jack Daniels will hang on.”
Quelle: Sky&Telescope