.
22.01.2015
COMET CLOSE-UPS
High-resolution images from ESA’s Rosetta spacecraft reveal an incredible array of surface features on the comet
This OSIRIS narrow-angle camera image shows part of a large fracture running across Comet 67P/Churyumov–Gerasimenko’s neck, in particular where it has left Hapi and is extending into Anuket. In this orientation, the Seth region is at the uppermost left and Hapi in the lower left.
Credits: ESA/Rosetta/MPS for OSIRIS Team MPS/UPD/LAM/IAA/SSO/INTA/UPM/DASP/IDA
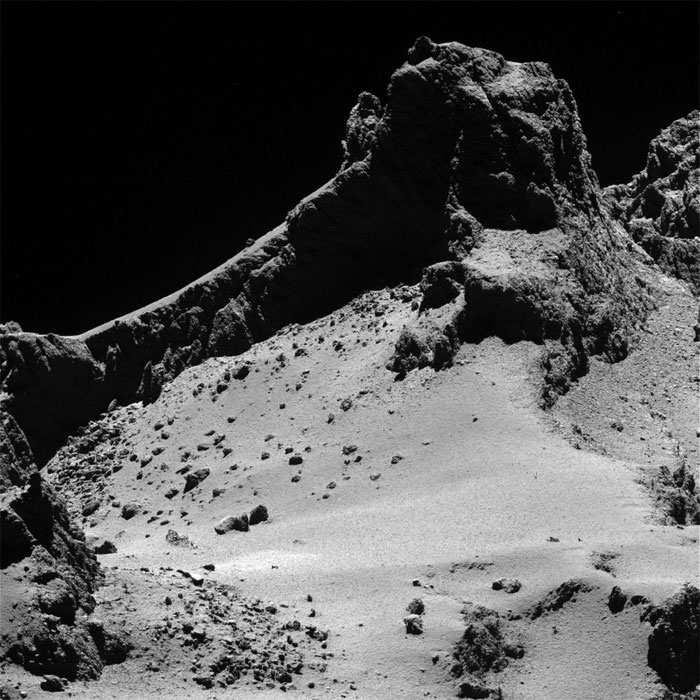
A section of the smaller of Comet 67P/Churyumov–Gerasimenko’s two lobes as seen through Rosetta’s narrow-angle camera from a distance of about 8 km to the surface on 14 October 2014. The resolution is 15 cm/pixel. The image is featured on the cover of 23 January 2014 issue of the journal Science.
Credit: ESA/Rosetta/MPS for OSIRIS Team MPS/UPD/LAM/IAA/SSO/INTA/UPM/DASP/IDA
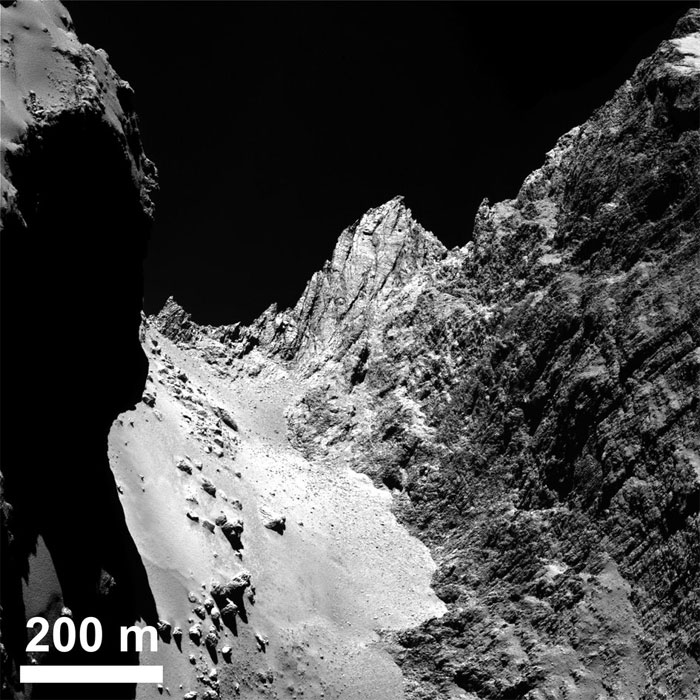
The boulder-strewn, smooth Hapi region in Comet 67P/Churyumov-Gerasimenko’s neck, with the Hathor cliff face to the right.
Credits: ESA/Rosetta/MPS for OSIRIS Team MPS/UPD/LAM/IAA/SSO/INTA/UPM/DASP/IDA
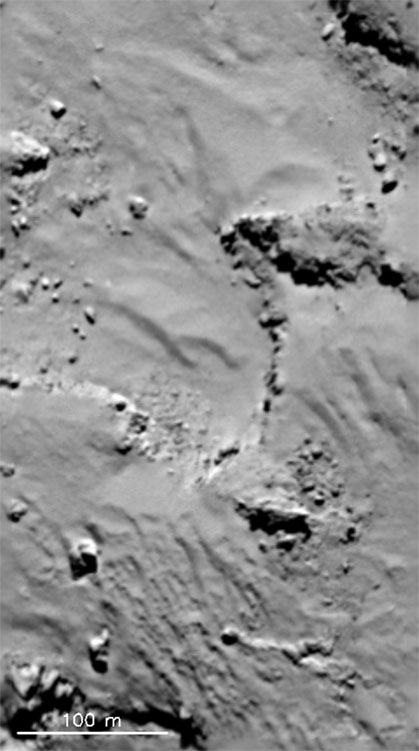
Evidence of dune-like structures in the surface dust of Comet 67P/Churyumov–Gerasimenko. Features like this may be the result of localised gas-driven transport of surface dust. The image was taken by the OSIRIS narrow-angle camera on 23 August 2014.
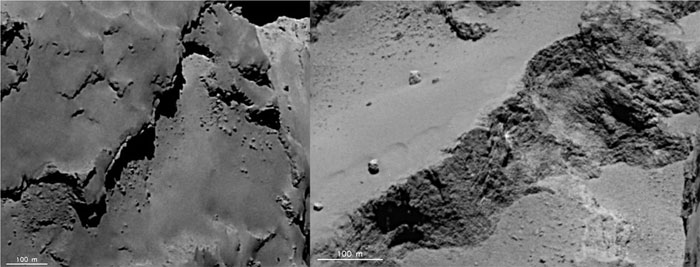
In this OSIRIS narrow-angle camera image the boundary between the Ash and Seth regions is highlighted. The left-hand image shows evidence of collapse along the boundary, with debris at the base of a cliff. Fracturing to produce crevices is also observed with ‘deflation’ of the surface appearing as a possible precursor to fracture or collapse. Surface deflation is also indicated in the right-hand image, above a fractured cliff face. The image also highlights the contrast between the dust covered horizontal slope and the ‘clean’ vertical face.
The left image was taken on 7 August 2014, the right image on 2 September 2014.
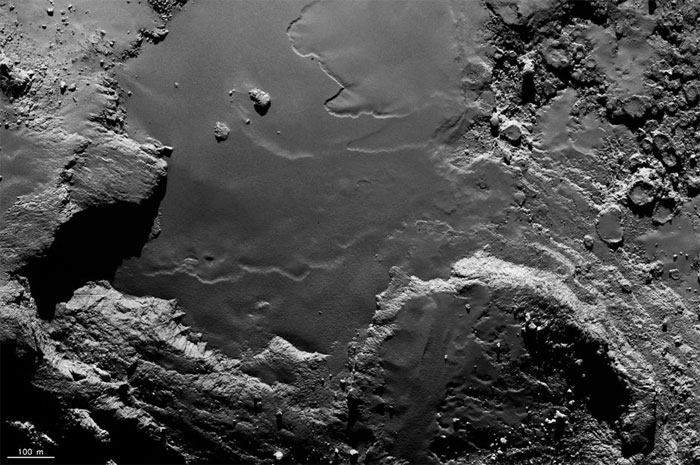
Part of the Imhotep region on Comet 67P/Churyumov–Gerasimenko’s large lobe; the image was taken by the OSIRIS narrow-angle camera on 5 September 2014. The region shows a wide range of complex terrain: smooth terrain, layering of this material at its margin, smooth material on topographically higher surfaces, circular structures, and layered consolidated material rising towards a 650 m-diameter raised semicircular structure. Fracturing of the consolidated material is evident throughout.
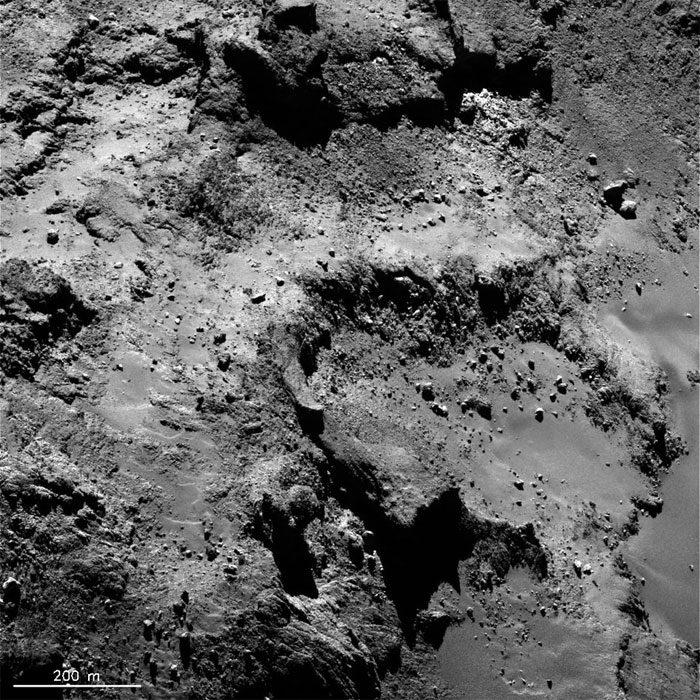
Surface textures on the border of the board, smooth region in Imhotep, on the large lobe of Comet 67P/Churyumov–Gerasimenko.
Credit: ESA/Rosetta/MPS for OSIRIS Team MPS/UPD/LAM/IAA/SSO/INTA/UPM/DASP/IDA
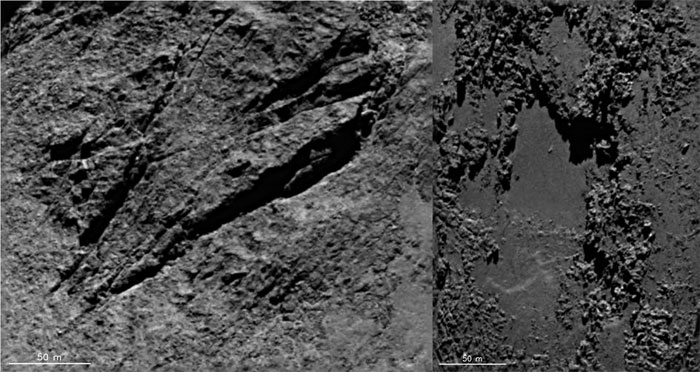
Left: Tectonic feature in the Aker region, imaged on 18 September 2014 with the OSIRIS narrow-angle camera.
Right: Ponded deposits in the Khepry region, imaged on 16 September 2014 with the OSIRIS narrow-angle camera.
Credits: ESA/Rosetta/MPS for OSIRIS Team MPS/UPD/LAM/IAA/SSO/INTA/UPM/DASP/IDA
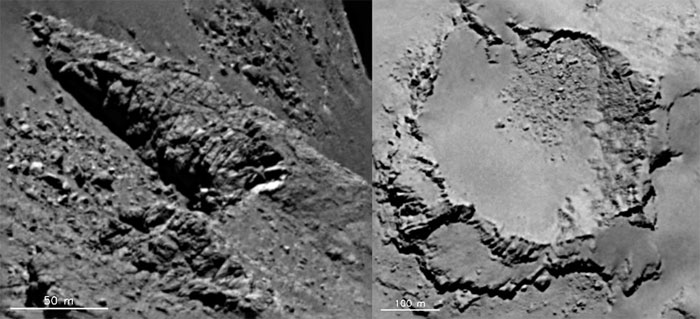
Left: an exposed spur at the interface to Hapi showing extensive irregular fracturing. The image was taken with the OSIRIS narrow-angle camera on 12 September 2014.
Right: circular depression that appears to have produced uplift with columnar jointing structures. Evidence of collapse and debris is also present. The image was taken with the OSIRIS narrow-angle camera on 8 August 2014.
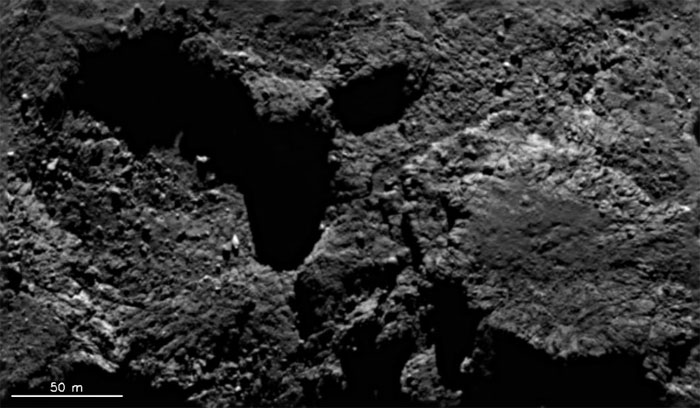
In the Maftet region, a block of material to the right has a similar shape and appearance to the depression on the left. The block is around 100 m in diameter, and the pit is around 150 m across. One theory is that this is evidence of surface disruption and material ejection produced by subsurface gas pressure build-up of a super-volatile such as carbon monoxide or carbon dioxide. However, the proposal needs to overcome the high porosity of the nucleus and explain how gas can be trapped in a pocket so that pressure can build. Mechanisms producing subsurface ice barriers (through volatile transport to colder areas within the nucleus) need to be considered.
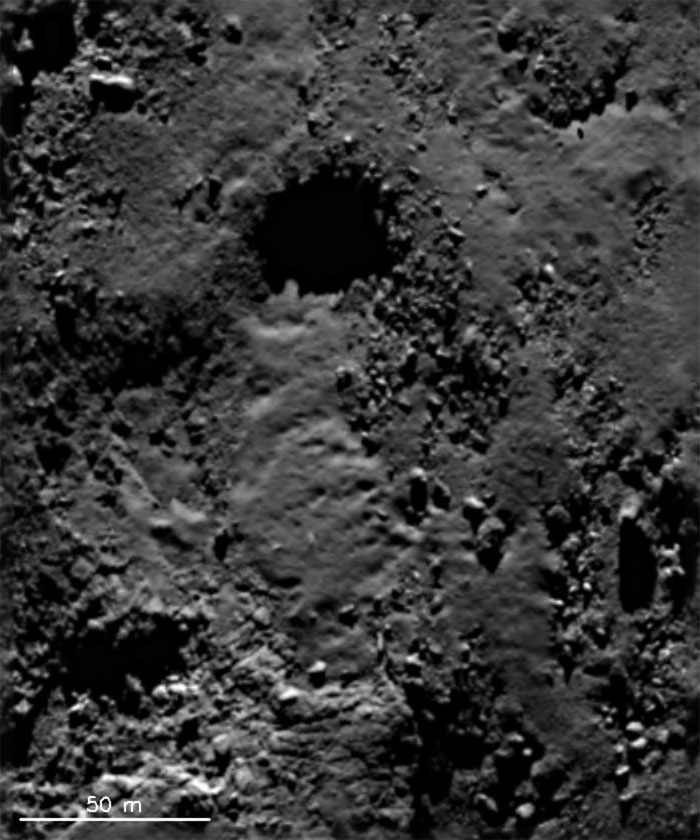
This image, captured on 19 September 2014, provides evidence of material flowing out from a pit in the Maftet region of Comet 67P/Churyumov–Gerasimenko. The observation supports the concept of subsurface fluidisation mechanisms.
Credits: ESA/Rosetta/MPS for OSIRIS Team MPS/UPD/LAM/IAA/SSO/INTA/UPM/DASP/IDA
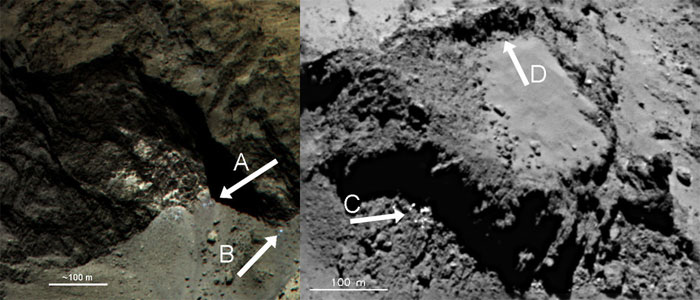
Left: Colour composite of an alcove at the Hathor–Anuket boundary. The surface of the consolidated material in the alcove contains distinct spots of brighter material. On the floor beneath, material 20% brighter and bluer than the surroundings can also be seen (e.g. A and B), and may be evidence of recently exposed ice. The colour image was created from the red, green and blue filters (central wavelengths of (882, 700 and 481 nm, respectively) of the OSIRIS narrow-angle camera and the image sequence was acquired on 21 August 2014.
Right: Bright, highly reflective metre‐size boulders (C) at the Ash–Khepry boundary. An example of brittle fracturing is indicated at D. The image was acquired on 3 September 2014 with the OSIRIS narrow-angle camera.
Credits: ESA/Rosetta/MPS for OSIRIS Team MPS/UPD/LAM/IAA/SSO/INTA/UPM/DASP/IDA
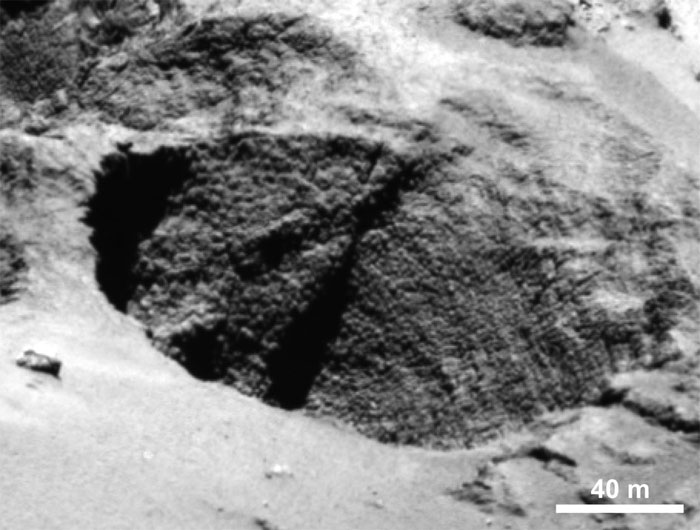
Close-up of a curious surface texture nicknamed ‘goosebumps’. The characteristic scale of all the bumps seen on Comet 67P/Churyumov–Gerasimenko by the OSIRIS narrow-angle camera is approximately 3 m, extending over regions greater than 100 m. They are seen on very steep slopes and on exposed cliff faces, but their formation mechanism is yet to be explained.
Credits: ESA/Rosetta/MPS for OSIRIS Team MPS/UPD/LAM/IAA/SSO/INTA/UPM/DASP/IDA
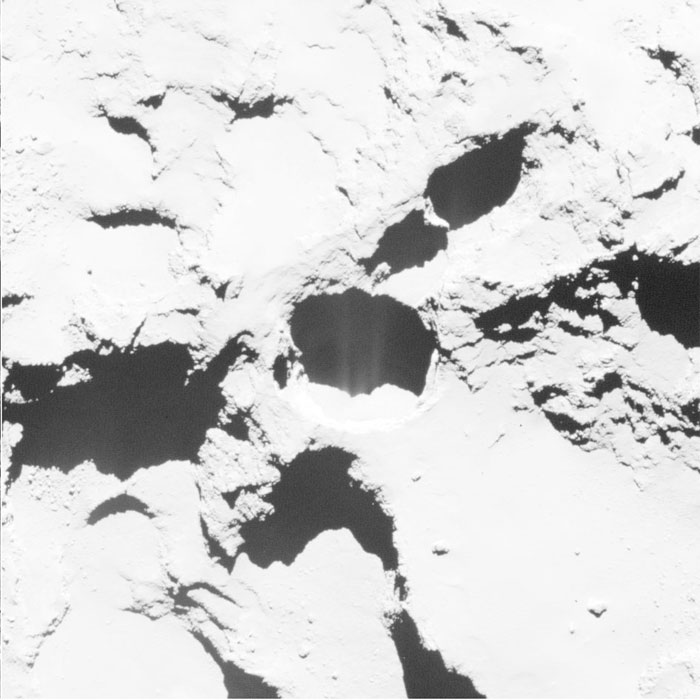
Enhanced contrast image of an active pit detected in Seth region of Comet 67P/Churyumov–Gerasimenko. The enhanced image reveals fine structures in the shadow of the pit, interpreted as jet-like features rising from the pit. This OSIRIS narrow-angle camera image was acquired on 28 August 2014 at a distance of 60 km from the comet. The image resolution is 1 m/pixel.
Credits: ESA/Rosetta/MPS for OSIRIS Team MPS/UPD/LAM/IAA/SSO/INTA/UPM/DASP/IDA
Quelle: ESA
---
COMET’S COMA COMPOSITION VARIES SIGNIFICANTLY OVER TIME
Rosetta scientists measuring the composition of comet 67P’s atmosphere or coma discovered that it varies greatly over time. Large fluctuations in composition in a heterogeneous coma indicate day-night and possibly seasonal variations in the major outgassing species: H2O, CO, and CO2. The red region where CO and CO2 dominate is a part of the comet that is poorly illuminated, indicating a complex coma-nucleus relationship where seasonal variations may be driven by temperature differences just below the comet surface.
Shape model credit: ESA/Rosetta/MPS for OSIRIS Team MPS/UPD/LAM/IAA/SSO/INTA/UPM/DASP/IDA
-
This entry is based on the Southwest Research Institute’s press release and covers the results of the ROSINA instrument – the Rosetta Orbiter Spectrometer for Ion and Neutral Analysis – that were published today in the journal Science.
Scientists working on the ROSINA instrument have discovered that Comet 67P/Churyumov-Gerasimenko’s atmosphere, or coma, is much less homogenous than expected and that comet outgassing varies significantly over time.
“If we would have just seen a steady increase of gases as we closed in on the comet, there would be no question about heterogeneity of the nucleus,” says Dr. Myrtha Hässig, lead author of the paper and a postdoctoral researcher at Southwest Research Institute in San Antonio. “Instead we saw spikes in water readings, and a few hours later, a spike in carbon dioxide readings. This variation could be a temperature effect or a seasonal effect, or it could point to the possibility of comet migrations in the early Solar System.”
“Our whole concept of the variability of volatile release at comets will change based on this paper, which will have significant impact on our understanding of comet formation and evolution,” says Dr. Hunter Waite, a program director and planetary scientist at SwRI.
Comets are small Solar System bodies with a nucleus composed of ice, dust, and small rocky particles. As comets approach the Sun along their orbit, they heat up and outgas, displaying visible atmospheres and tails. Comets contain some of the best-preserved material from the formation of our planetary system, offering clues about physical and chemical conditions that existed in the early Solar System.
Rosetta has been studying Comet 67P/C-G since it began approaching the four kilometre wide world last year, finally arriving at a distance of 100 km on 6 August 2014.
“From a telescope, images of a comet’s atmosphere suggest that the coma is uniform and does not vary over short periods of hours or days. That’s what we were expecting as we approached the comet,” said Dr. Stephen Fuselier, a director in the SwRI Space Science and Engineering Division and the lead U.S. co-investigator for ROSINA’s DFMS instrument. “It was certainly a surprise when we saw time variations from 200 km away. More surprising was that the composition of the coma was also varying by very large amounts. We’re taught that comets are made mostly of water ice. For this comet, the coma sometimes contains much more carbon dioxide than water vapour.”
Indeed, ROSINA data indicate that the H2O signal is strongest overall; however, there are periods when the CO and CO2 rival that of H2O.
“These large fluctuations in composition in a heterogeneous coma indicate diurnal or day-night and possibly seasonal variations in the major outgassing species,” says Hässig. “When I first saw this behavior, I thought something may have been wrong, but after triple-checking the data, we believe 67P has a complex coma-nucleus relationship, with seasonal variations possibly driven by temperature differences just below the comet surface.”
The nucleus of 67P/C-G consists of two lobes of different sizes, connected by a neck region. This complex, “rubber-ducky” shape likely plays a key role in this variation, as different portions of the nucleus face the Sun during the comet’s 12.4 rotation cycle. If the coma composition reflects the composition of the nucleus, variations suggest that the nucleus may have formed by collision of smaller bodies that originated from very different regions of the early Solar System. This discovery could revolutionize theories about comet formation and the relationship between comet composition and the location of its origin.
As the comet continues its 6.5-year journey around the Sun, Rosetta scientists will be able to consider seasonal variations as well.
“Time Variability and Heterogeneity in the Coma of 67P/Churyumov-Gerasimenko” is published in the 23 January issue of the journal Science.
The ROSINA team is led by Kathrin Altwegg of the University of Bern, Switzerland.
---
GIADA’S DUST MEASUREMENTS: 3.7-3.4 AU
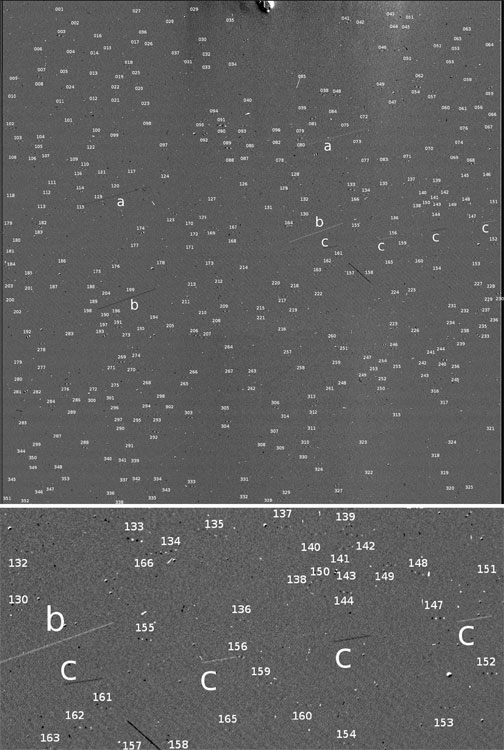
Bound grains detected in the first set of OSIRIS NAC images (each labelled by a from 001 to 353) and the brightest 3 tracks of the 48 out-flowing grains (labelled by the letters a, b and c, with the track of c shown in more detail in the bottom image). The detections of bound grains (white-black-white-black dots) show a uniform space distribution. The comet nucleus is visible at top of the image. Credit: ESA/Rosetta/MPS for OSIRIS Team MPS/UPD/LAM/IAA/SSO/INTA/UPM/DASP/IDA
-
Based on inputs from GIADA team members Alessandra Rotundi (instrument PI) and Marco Fulle, following the publication of “Dust Measurements in the Coma of Comet 67P/Churyumov-Gerasimenko Inbound to the Sun Between 3.7 and 3.4 AU” in the journal Science today. GIADA is Rosetta’s Grain Impact Analyzer and Dust Accumulator.
Our published research so far focuses on two aspects of the dust around Comet 67P/C-G: the different populations of dust grain found either in bound orbits or out-flowing from the nucleus, and the dust-to-gas ratio in the coma.
First let’s discuss the grain population, considering measurements made with both GIADA and OSIRIS on 4 August 2014, when we were still at 275 km from the comet. These observations allowed us to count about 350 grains in bound orbits around the comet nucleus, and 48 fast, out-flowing grains that were ejected about a day before the observations. These two families of detected grains – out-flowing and bound – do not overlap in space. Out-flowing grains were not detected farther than 20 km from the spacecraft, whereas bound grains were not detected closer than 130 km from the spacecraft (that is, they were found within about 145 km of the comet).
The space density of bound grains is at least 100 times lower than that of out-flowing grains and, in general bound grains are much bigger than out-flowing grains. Indeed, based on the observed brightness range, we infer that the bound grains varied from 4 cm to 2 m*, whereas the out-flowing grains seen in the images were less than 1.7 cm. And in fact the largest grain detected directly by GIADA is on the order of 0.1mm. We don’t see so many larger grains outflowing from the comet, because the gas density at the nucleus surface was still unable to lift larger grains at these distances from the Sun (about 553 million km to 508 million km).
(*Editor’s note: In a follow-up discussion, I asked Alessandra and Marco about the possible impact – literally – of ‘grains’ up to 2 metres in size on the spacecraft, but there is no need to panic: according to their space density, Rosetta needs to travel for many centuries at a speed of 1 m/s before impacting even just one of them!)
While we expect more outflowing grains as we get ever closer to perihelion in August, the bound grains also have an interesting story to tell. We think they are left over from the comet’s last excursion around the Sun, left suspended around the comet after the gas flow had decreased such that it could no longer perturb the orbits. The number and range of size of dust particle observed requires several years to build up, so they could not be ejected during the outburst observed at the end of April 2014, for example – all of that dust escaped into the tail of the comet without any collision with the bound grains.
Will the cloud dissipate once activity increases? Well, this is an example of where orbiting very close to the comet actually puts us at a disadvantage! Even at 30 km distance the images are so full of out-flowing grains, that to identify the few hundreds of tiny dots of the far bound grains is practically impossible. We’ll have to wait a few more months to check in on this population when we are flying further from the comet nucleus, when conditions will be again favourable to the detection of bound grains.
Dust-to-gas ratio
Now let’s look at the dust-to-gas ratio. By considering data from GIADA, OSIRIS, MIRO and ROSINA, this was determined to be 4 ± 2 for Comet 67P/C-G. Why should we care about this ratio and what does it really mean? Well, it provides important information about where comets formed and how they evolve – it actually helps us to say how much the classical view of a "dirty snowball" is correct. In that case, we’d expect a dust-to-gas ratio in the range of 0.1-1, so you can see that really they are more like “snowy dustballs” than dirty snowballs! For another comparison, if we assume only water is being emitted from the comet then the derived dust:gas ratio would be 6 ± 2. This ratio decreases to 4 ± 2 if we include CO and CO2.
The dust-to-gas ratio is also important to infer the internal porosity. Since dust has a bulk density larger than ice, the larger the dust-to-gas ratio, the larger the internal nucleus porosity we need to explain the measured low bulk density of the nucleus.
An estimated value of 3 has been made for comet 67P/C-G at perihelion, so we’re looking forward to seeing if/how the value changes in the coming months.
It has the potential to change because the value reflects an average composition of the material being lost from the nucleus, which currently is very dusty. The value also assumes that we can separate solids (dust) from volatiles/ices (gas). We use the GIADA data to check the bulk density of the grains so that any ice-rich grains would be excluded from the dust count. But, at the time of the observations at least, the out-flowing grains are very small (less than 2 cm as we discussed in the previous section). In grains of these sizes, ice would take very little time to sublimate. When the comet starts to eject bigger clumps (metre-sized clumps at next perihelion, as already observed in the bound cloud as being remnants from the last perihelion), then the ice content in these clumps could contribute to the gas flux, and so will have to be taken into account when we calculate the ratio again in August.
It’s a complicated and ever-changing story!
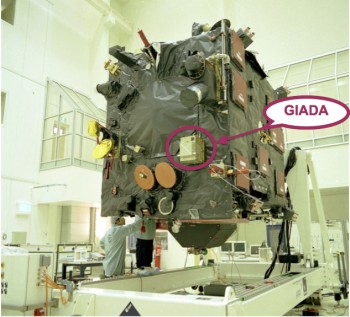
The location of GIADA marked on the Rosetta spacecraft.
Image courtesy Alessandra Rotundi
---
WATCHING THE BIRTH OF A COMET MAGNETOSPHERE
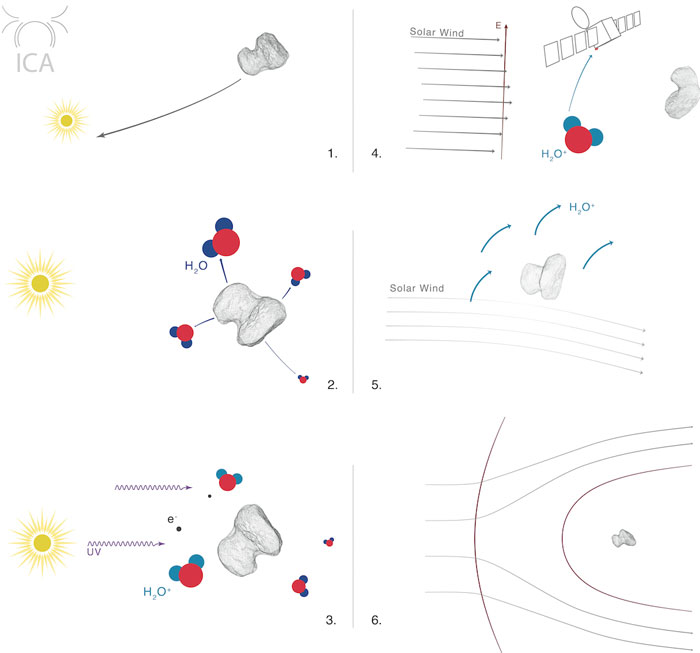
How a comet grows a magnetosphere
1. The comet approaches the Sun
2. Water molecules sublimate from the comet as it thaws
3. The water molecules are ionised by ultraviolet light from the Sun
4. Newborn ions are accelerated by the solar wind electric field and are detected by the RPC-ICA instrument
5. The solar wind accelerates the water ions in one direction, but is itself deflected in the opposite direction
Credits: ESA/Rosetta/RPC-ICA
-
Based on the press release of the Swedish Institute of Space Physics summarising the results of the Rosetta Plasma Consortium’s (RPC) Ion Composition Analyser (ICA) that are presented in the journal Science today, and on follow-up discussion with Hans Nilsson, RPC-ICA principal investigator.
The RPC-ICA instrument onboard Rosetta has been watching the early stages of how a magnetosphere forms around Comet 67P Churyumov-Gerasimenko as it moves closer to the Sun along its orbit and begins to interact with the solar wind.
As the comet gets warmer, volatile substances, mainly water, evaporate from the surface and form an atmosphere around the comet. The Sun's ultraviolet radiation and collisions with the solar wind ionizes some of the comet's atmosphere. The newly formed ions are affected by the solar wind electric and magnetic fields and can be accelerated to high speeds. When the comet gets close enough to the Sun, its atmosphere becomes so dense and ionized that it becomes electrically conductive. When this happens, the atmosphere starts to resist the solar wind and a comet's magnetosphere is born – a region surrounding the comet that is shielded from the solar wind.
"The comet environment is a laboratory for scientists; we can see what happens when the solar wind streams through an atmosphere," says Associate Professor Hans Nilsson at the Swedish Institute of Space Physics (IRF) in Kiruna. Hans Nilsson is responsible for the instrument Ion Composition Analyser, ICA, developed and built in Kiruna. The instrument measures positively charged ions and is one of five instruments in the Rosetta Plasma Consortium.
Hans explained that studying the interaction of the solar wind with a comet's atmosphere in this way could shed light on how the solar wind interacted with the planets during their early formation. "In the young Solar System, the planet’s upper atmospheres were strongly heated and extended far into space. The solar wind should have streamed through the outer part of these extended atmospheres in a way that resembles its passage through the comet atmosphere for this low activity stage," he explains.
At 67P/C-G, Rosetta’s RPC-ICA instrument detected low velocity water ions in data collected on 7 August 2014, one day after arriving at the comet at a distance of 100 km.
"It was an unambiguous signature of the comet, a clear detection of ions from the comet's atmosphere," confirmed Hans.
Rosetta is a unique space mission. Previous spacecraft that have studied comets have rushed past them at a great distance and at speeds of tens of kilometres per second. At the time of these previous spacecraft-comet encounters, the comet magnetosphere had been fully developed. By comparison, Rosetta is currently orbiting around 67P/C-G at a distance of just a few tens of kilometres, and at low speed. Importantly, the mission arrived at the comet in time to watch the very early stages of the magnetosphere formation.
“For the first time, we can see what happens before the comet atmosphere resists the solar wind," says Hans. "We discovered that the comet atmosphere affects the solar wind more than we thought it would at this early stage. We are also surprised how much structure we see in our data – the comet atmosphere appears to be very unevenly distributed around the nucleus.
"We are still in the early stages of analysing and modelling our data, but perhaps the energy transfer from the solar wind to the atmosphere is less efficient in removing an atmosphere than we originally thought.
As the comet moves closer to the Sun along its orbit, Hans and his colleagues will see the transition from this early phase to the growth of a well established comet magnetosphere.
"That transition is likely the most exciting part," he says. "It can happen any day now. Every morning we keep looking at the new data which have just arrived. What will we find today?"
"Birth of a comet magnetosphere: a spring of water ions," by Hans Nilsson et al., is published in 23 January issue of Science
---
Editor's note: On previous blog entries, some of you have been discussing about the formation of the comet's bow shock – a question I put to Hans, who says:
"We do not know for sure at what distance the bow shock is formed. At the distance we are right now [30 km from the comet] we can see that the solar wind is not shocked, and it does still permeate the comet atmosphere down to where Rosetta is located. So we do know that the magnetosphere has not formed yet, and we would at least indirectly see when it is formed. According to the models, the bow shock should form quite far away, so we would need rather large excursions away from the comet to observe it in situ."
Quelle: ESA
---
GETTING TO KNOW ROSETTA’S COMET – SCIENCE SPECIAL EDITION
In a special edition of the journal Science, published online tonight, results are presented from seven of Rosetta’s 11 science instruments made during the approach to and soon after arriving at Comet 67P/Churyumov-Gerasimenko in August 2014.
Our overview article is published on the main ESA Portal and also posted below. A number of supporting blog posts provided by the different instrument teams to give more details of their specific results will also soon be published on the blog. In addition, we are pleased to point you to our “Comet close-ups” image slideshow that focuses on the OSIRIS images presented in the papers – enjoy!
.
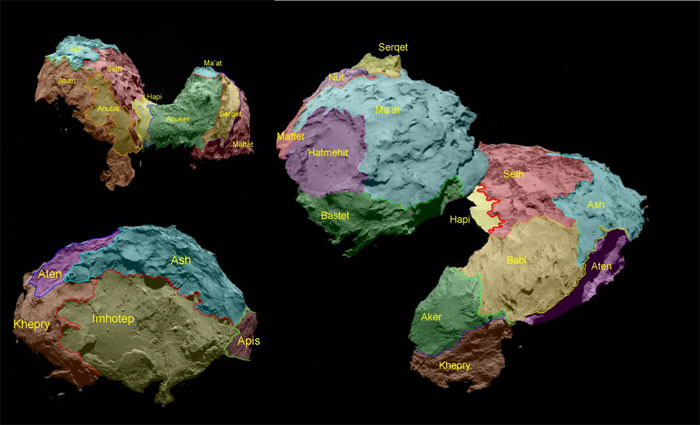
Comet regional maps. Credit: ESA/Rosetta/MPS for OSIRIS Team MPS/UPD/LAM/IAA/SSO/INTA/UPM/DASP/IDA
.
Getting to know Rosetta’s comet
Rosetta is revealing its host comet as having a remarkable array of surface features and with many processes contributing to its activity, painting a complex picture of its evolution.
-
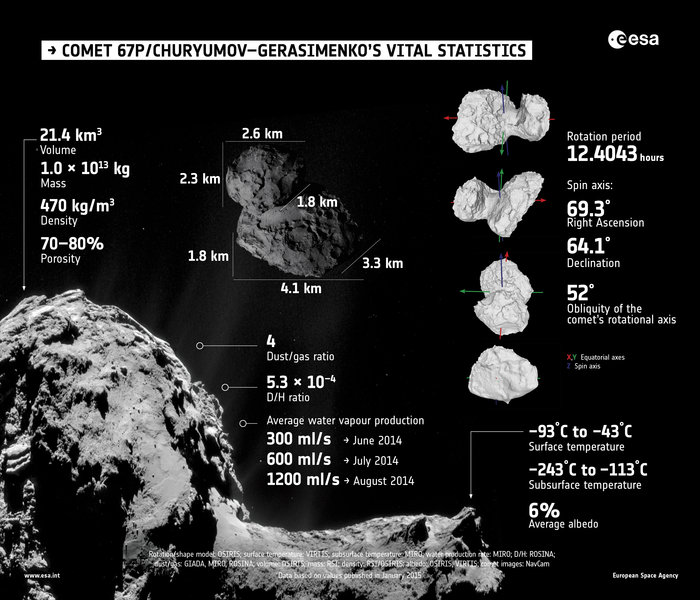
The familiar shape of the dual-lobed comet has now had many of its vital statistics measured: the small lobe measures 2.6 × 2.3 × 1.8 km and the large lobe 4.1 × 3.3 × 1.8 km. The total volume of the comet is 21.4 km^3 and the Radio Science Instrument has measured its mass to be 10 billion tonnes, yielding a density of 470 kg/m^3.
By assuming an overall composition dominated by water ice and dust with a density of 1500–2000 kg/m3, the Rosetta scientists show that the comet has a very high porosity of 70–80%, with the interior structure likely comprising weakly bonded ice-dust clumps with small void spaces between them.
The OSIRIS scientific camera, has imaged some 70% of the surface to date: the remaining unseen area lies in the southern hemisphere that has not yet been fully illuminated since Rosetta’s arrival.
The scientists have so far identified 19 regions separated by distinct boundaries and, following the ancient Egyptian theme of the Rosetta mission, these regions are named for Egyptian deities, and are grouped according to the type of terrain dominant within.
Five basic – but diverse – categories of terrain type have been determined: dust-covered; brittle materials with pits and circular structures; large-scale depressions; smooth terrains; and exposed more consolidated (‘rock-like’) surfaces.
Much of the northern hemisphere is covered in dust. As the comet is heated, ice turns directly into gas that escapes to form the atmosphere or coma. Dust is dragged along with the gas at slower speeds, and particles that are not travelling fast enough to overcome the weak gravity fall back to the surface instead.
Some sources of discrete jets of activity have also been identified. While a significant proportion of activity emanates from the smooth neck region, jets have also been spotted rising from pits.
The gases that escape from the surface have also been seen to play an important role in transporting dust across the surface, producing dune-like ripples, and boulders with ‘wind-tails’ – the boulders act as natural obstacles to the direction of the gas flow, creating streaks of material ‘downwind’ of them.
The dusty covering of the comet may be several metres thick in places and measurements of the surface and subsurface temperature by the Microwave Instrument on the Rosetta Orbiter, or MIRO, suggest that the dust plays a key role in insulating the comet interior, helping to protect the ices thought to exist below the surface.
Small patches of ice may also be present on the surface. At scales of 15–25 m, Rosetta’s Visible, InfraRed and Thermal Imaging Spectrometer, or VIRTIS, finds the surface to be compositionally very homogenous and dominated by dust and carbon-rich molecules, but largely devoid of ice. But smaller, bright areas seen in images are likely to be ice-rich. Typically, they are associated with exposed surfaces or debris piles where collapse of weaker material has occurred, uncovering fresher material.
On larger scales, many of the exposed cliff walls are covered in randomly oriented fractures. Their formation is linked to the rapid heating–cooling cycles that are experienced over the course of the comet’s 12.4-hour day and over its 6.5-year elliptical orbit around the Sun. One prominent and intriguing feature is a 500 m-long crack seen roughly parallel to the neck between the two lobes, although it is not yet known if it results from stresses in this region.
Some very steep regions of the exposed cliff faces are textured on scales of roughly 3 m with features that have been nicknamed ‘goosebumps’. Their origin is yet to be explained, but their characteristic size may yield clues as to the processes at work when the comet formed.
And on the very largest scale, the origin of the comet’s overall double-lobed shape remains a mystery. The two parts seem very similar compositionally, potentially favouring the erosion of a larger, single body. But the current data cannot yet rule out the alternative scenario: two separate comets formed in the same part of the Solar System and then merged together at a later date. This key question will be studied further over the coming year as Rosetta accompanies the comet around the Sun.
How to grow an atmosphere
Their closest approach to the Sun occurs on 13 August at a distance of 186 million kilometres, between the orbits of Earth and Mars. As the comet continues to move closer to the Sun, an important focus for Rosetta’s instruments is to monitor the development of the comet’s activity, in terms of the amount and composition of gas and dust emitted by the nucleus to form the coma.
Images from the scientific and navigation cameras have shown an increase in the amount of dust flowing away from the comet over the past six months, and MIRO showed a general rise in the comet’s global water vapour production rate, from 0.3 litres per second in early June 2014 to 1.2 litres per second by late August. MIRO also found that a substantial portion of the water seen during this phase originated from the comet’s neck.
Water is accompanied by other outgassing species, including carbon monoxide and carbon dioxide. The Rosetta Orbiter Spectrometer for Ion and Neutral Analysis, ROSINA, is finding large fluctuations in the composition of the coma, representing daily and perhaps seasonal variations in the major outgassing species. Water is typically the dominant outgassing molecule, but not always.
By combining measurements from MIRO, ROSINA and GIADA (Rosetta’s Grain Impact Analyzer and Dust Accumulator) taken between July and September, the Rosetta scientists have made a first estimate of the comet’s dust-to-gas ratio, with around four times as much mass in dust being emitted than in gas, averaged over the sunlit nucleus surface.
However, this value is expected to change once the comet warms up further and ice grains – rather than pure dust grains – are ejected from the surface.
GIADA has also been tracking the movement of dust grains around the comet, and, together with images from OSIRIS, two distinct populations of dust grains have been identified. One set is outflowing and is detected close to the spacecraft, while the other family is orbiting the comet no closer than 130 km from the spacecraft.
It is thought that the more distant grains are left over from the comet’s last closest approach to the Sun. As the comet moved away from the Sun, the gas flow from the comet decreased and was no longer able to perturb the bound orbits. But as the gas production rate increases again over the coming months, it is expected that this bound cloud will dissipate. However, Rosetta will only be able to confirm this when it is further away from the comet again – it is currently in a 30 km orbit.
As the gas–dust coma continues to grow, interactions with charged particles of the solar wind and with the Sun’s ultraviolet light will lead to the development of the comet’s ionosphere and, eventually, its magnetosphere. The Rosetta Plasma Consortium, or RPC, instruments have been studying the gradual evolution of these components close to the comet.
“Rosetta is essentially living with the comet as it moves towards the Sun along its orbit, learning how its behaviour changes on a daily basis and, over longer timescales, how its activity increases, how its surface may evolve, and how it interacts with the solar wind,” says Matt Taylor, ESA’s Rosetta project scientist.
“We have already learned a lot in the few months we have been alongside the comet, but as more and more data are collected and analysed from this close study of the comet we hope to answer many key questions about its origin and evolution.”
These are among the very first scientific results from Rosetta and there is much more to come as the scientists work through the data and as the comet continues to evolve during its closest approach to the Sun. They are described in more detail in accompanying posts (to be published after this post and tomorrow) and in the 23 January 2015 Science special edition (reference below)
Quelle: ESA
18096 Views