.
The first 90-day run of the Large Underground Xenon (LUX) experiment showed the detector to be the most sensitive in the world. The experiment did not detect dark matter particles during its initial run, but it has ruled out “possible” findings elsewhere. The research team will fine-tune the detector’s sensitivity and begin a 300-day run in 2014.
PROVIDENCE, R.I. [Brown University] — In its first three months of operation, the Large Underground Xenon (LUX) experiment has proven itself to be the most sensitive dark matter detector in the world, scientists with the experiment announced today.
.
“LUX is blazing the path to illuminating the nature of dark matter,” Rick Gaitskell, professor of physics at Brown University and co-spokesperson for LUX. The detector’s location, more than a mile underground at the Sanford Underground Research Facility in South Dakota, offers a “supremely quiet” environment to detect the rare, weak interactions between dark matter particles and ordinary matter, Gaitskell said.
.
The first results from the experiment’s initial 90-day run were announced today during a seminar at the Sanford Lab in Lead, S.D.
“What we’ve done in these first three months of operation is look at how well the detector is performing, and we’re extremely pleased with what we’re seeing,” said Gaitskell, one of the founders of the LUX experiment. “This first run demonstrates a sensitivity that is better than any previous experiment looking to detect dark matter particles directly.”
.
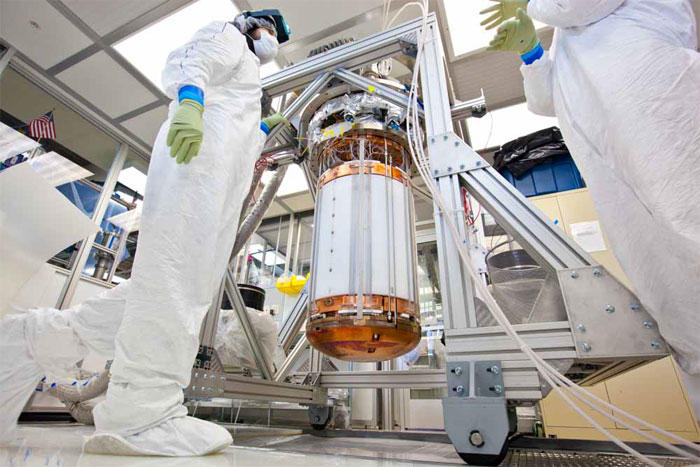
How to catch a WIMP
Photomultiplier tubes capable of detecting a single photon of light line the top and bottom of the LUX dark matter detector. They will record the position and intensity of collisions between dark matter particles and xenon nuclei. Credit: Photos by Matt Kapust/Sanford Underground Research Facility
.
With LUX’s initial run complete, the team will now make a few adjustments to fine-tune the device’s sensitivity in anticipation of a new 300-day run to begin in 2014.
Dark matter is thought to account for as much as 85 percent of the matter in the universe. But because it rarely interacts with other forms of matter, it has yet to be detected directly. The leading candidates for dark matter particles are called weakly interacting massive particles — WIMPS.
Theory and experimental results suggest that WIMPs could take either a high-mass or low-mass form. In the search for high-mass WIMPs weighing 40 times the mass of a proton, LUX has twice the sensitivity of any other dark matter direct-detection experiment, according to these new results. LUX also has greatly enhanced sensitivity to low-mass WIMPs, and new results suggest that potential detections of low-mass WIMPS by other dark matter experiments were likely the result of background radiation, not dark matter.
.
“There have been a number of dark matter experiments over the last few years that have strongly supported the idea that they’re seeing events in the lowest energy bins of their detectors that could be consistent with the discovery of dark matter,” Gaitskell said. “With the LUX, we have worked very hard to calibrate the performance of the detector in these lowest energy bins, and we’re not seeing any evidence of dark matter particles there.”
In the upcoming 300-day run, the LUX researchers hope either to detect dark matter definitively or to rule out a vast swath of parameter space where it might be found.
“Every day that we run a detector like this we are probing new models of dark matter,” Gaitskell said. “That is extremely important because we don’t yet understand the universe well enough to know which of the models is actually the correct one. LUX is helping to pin that down.”
.
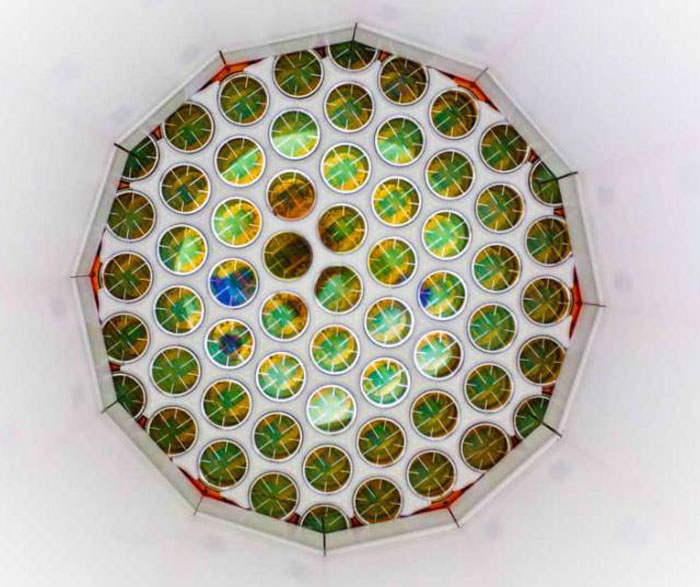
Separating signal from noise
The LUX detector is lined with white teflon to better gather faint signals of light that will be recorded by the photomultiplier tubes. Water — 70,000 gallons of it — provides further protection from background radiation.
.
Elusive particles
Though dark matter has not yet been detected directly, scientists are fairly certain that it exists. Without its gravitational influence, galaxies and galaxy clusters would simply fly apart into the vastness of space. But because dark matter does not emit or reflect light, and its interactions with other forms of matter are vanishingly rare, it is exceedingly difficult to spot.
“To give some idea of how small the probability of having a dark matter particle interact, imagine firing one dark matter particle into a block of lead,” Gaitskell said. “In order to get a 50-50 chance of the particle interacting with the lead, the block would need to stretch for about 200 light years — this is 50 times farther than the nearest star to the Earth aside from the sun. So it’s an incredibly rare interaction.”
Capturing those interactions requires an incredibly sensitive detector. The key part of the LUX is a third of a ton of supercooled xenon in a tank festooned with light sensors, each capable of detecting a single photon at a time. When a particle interacts with the xenon, it creates a tiny flash of light and an ion charge, both of which are picked up by the sensors.
To minimize extraneous interactions not due to dark matter, the detector must be shielded from background radiation and cosmic rays. For that reason, the LUX is located 4,850 feet underground, submerged in 71,600 gallons of pure de-ionized water.
But even in that fortress of solitude, occasional background interactions still happen. It’s the job of LUX physicists to separate the signal from the noise.
Watching interactions, one by one
During its initial run, the LUX picked up xenon flashes in the energy region of interest for dark matter at a rate of about one per day. By looking carefully at the nature of each interaction, the researchers can tell which ones are from residual background radiation and which could be due to dark matter.
“Dark matter will interact with the nucleus of xenon atom, while most forms of radioactive background tend to interact with the outer electrons,” Gaitskell explained. “Each of those interactions produces a recoil, either of the nucleus or the electrons. So at the rate of about one a day, we see these interactions and test to see if they are consistent with a nuclear recoil or an electron recoil. So far every event we have seen has looked like a conventional electromagnetic background event.”
But as the detector runs for longer periods, the odds that a dark matter interaction will be captured increase. And the LUX, says Gaitskell, has the sensitivity to catch it.
“LUX is a huge step forward. Within the first few minutes of switching it on, we surpassed the sensitivity of the first dark matter detectors I was involved with 25 years ago,” he said. “Within a few days, it surpassed the sensitivity of sum total of all previous dark matter direct search experiments I have ever worked on. This first LUX run is more sensitive than any previous search conducted and now sets us up perfectly for the 300-day run to follow.”
.
Collaborative effort
The LUX scientific collaboration includes 17 research universities and national laboratories in the United States, the United Kingdom, Portugal, and Russia. The work is supported by the U.S. Department of Energy and the State of South Dakota. At Brown, 18 postdoctoral researchers, graduate students, and undergraduates have worked on the project.
Brown University research associate Simon Fiorucci, postdoctoral researcher Monica Pangilinan, and graduate students Jeremy Chapman, David Malling, and Carlos Faham have been working on the LUX project since its inception. Fiorucci is now the science coordination manager for the project and has been instrumental in delivering the new science. Chapman and Malling’s Brown University theses contain the primary analysis used for this latest result. “We are very excited that our thesis work has culminated in this world-leading result,” Chapman said.
“Support from the administration at Brown was instrumental in the LUX getting the experiment off the ground and enabling us to maintain a leading position in the experiment.” Gaitskell said. “The Brown group has been extremely important to making this experiment be the success that it is.”
.
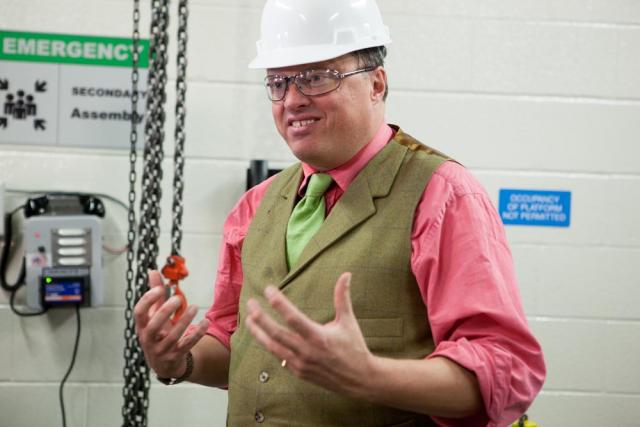
Physicist Rick Gaitskell
“Every day that we run a detector like this we are probing new models of dark matter. ... We don’t yet understand the universe well enough to know which of the models is actually the correct one.”
.
Quelle: Brown University
.
New dark matter detector sends first data from gold mine 1.5km underground
Scientists testing the Large Underground Xenon (LUX) experiment have reported promising scientific and technological results today.
They have set up the experiment to identify the nature of dark matter, an invisible substance that physicists believe is all around us, making up most of the matter in the universe, but that barely has any effect on our every-day lives.
Scientists have published the first results from the Sanford laboratory today, which, they say, validate the experiment's design and performance. The research challenges previous studies that claim 'sightings' of dark matter.
They are now beginning a process to uncover the exact identity of the dark matter particle - a process equivalent to the work done by the Large Hadron Collider in identifying the Higgs boson.
Seventeen universities and research institutes in the USA and Portugal, and Imperial College London, UCL, University of Edinburgh in the UK, run the LUX experiment, with most funding coming from the National Science Foundation and Department of Energy in the USA.
The new laboratory is sited in a former gold mine nearly one and a half kilometres below the Black Hills in the American state of South Dakota. Work on LUX started in 2008 and the experiment was completed ready for an initial run earlier in 2013.
Here, scientists are operating some of the world's most sensitive equipment in an extremely sheltered environment, because they are looking for tiny and extremely rare flashes of light that would indicate a collision between a dark matter particle and a normal matter particle.
"These instruments take many years to build and we are always pushing new technologies to the limit," says Dr Henrique Araújo from the Department of Physics at Imperial College London who leads the College's team working on LUX.
"It is very significant that LUX worked as designed when we finally pushed the 'on' button. Many experiments never reach this stage."
Physicists believe that dark matter accounts for about a quarter of the energy in the Universe, compared with ordinary matter, which makes up only a twentieth. The rest consists of the even more mysterious dark energy.
Since the experiment was installed underground in February, they have been looking for Weakly Interacting Massive Particles (WIMPs), which are the prime candidates to constitute the dark matter in our galaxy and in the Universe.
These particles are thought to have mass like normal particles and create a tiny gravitational pull, but cannot be observed directly since they neither emit nor absorb light at any wavelength. On the largest scales, its presence can be inferred from the motion of stars within galaxies, and of individual galaxies in galactic clusters.
Collisions between WIMPs and normal matter are rare and extremely difficult to detect because cosmic-ray particles from space can overwhelm the already faint flashes expected from WIMPs.
Few cosmic rays can penetrate as deep underground as the LUX experiment and the detector is further protected from background radiation by being immersed in a shielding tank of ultra-pure water.
"We are able to detect the faint flashes of light very effectively using good internal reflector materials and very sensitive photon sensors," says Dr Araújo.
"LUX has significantly higher sensitivity than the previous world's best dark matter experiments – especially for the lightest WIMPs, which cause the faintest signals."
The new LUX result challenges evidence from other experiments, such as CoGeNT and DAMA, where scientists have previously claimed to have data about the nature of WIMPs.
Dr Araújo says: "A number of previous results make it look like WIMPs exist with a particularly low mass. While this may still turn out to be the case, our new data reveal that, on that occasion, it was a case of mistaken identity."
A decade ago, scientists of the UK-led ZEPLIN programme deployed the first dark matter detector of this type underground at the Boulby mine in North Yorkshire.
"We had a pioneering role in what has become the world's most sensitive dark matter search technology, building and operating three detectors at Boulby."
"The last and most sensitive, ZEPLIN-III, was built by our team at Imperial – until we concluded our programme in 2011 – joining LUX soon after."
Scientists including Dr Araújo are already designing – and soon will start building – the next-generation experiment, LZ, which is the coming together of the two programmes – LUX and ZEPLIN.
With a 7-tonne liquid xenon target, LZ will be 30 times larger than LUX and have over 100 times better range. It will be so sensitive that it will be limited only by the interference of background signals from astrophysical neutrinos. These similarly illusive particles were once a candidate to explain the dark matter problem — but physicists now know they are not massive enough to do the job.
LZ is a collaboration of 26 institutes in the US, UK, Portugal and Russia. Dr Araújo from Imperial leads the UK team on LZ, which counts also with colleagues from Edinburgh, UCL, Oxford and Sheffield universities as well as the Rutherford Appleton and Daresbury national laboratories.
.
Quelle: Imperial College London, South Kensington Campus
5677 Views